Introduction and Applications of Metal
Metals are substances that form naturally beneath the Earth's surface. Most metals are lustrous or shiny. Metals are inorganic, meaning they are composed of substances that were never alive.
Metals are a class of materials that are typically hard, shiny, malleable, ductile, and good conductors of heat and electricity. They make up about 75% of the elements in the periodic table and have been used by humans for thousands of years. Metal is very strong and durable, which is why it is used to make a wide variety of products. Metals are commonly used in automobiles, satellites, cooking utensils, and more.
Most metals are hard, but some are not. Sodium and potassium, for example, are metals that can be cut with a knife, while mercury is a liquid metal at room temperature. Iron, on the other hand, is solid.
Physical Properties of Metals
-
All metals are good conductors of heat and electricity. For example, cooking utensils and irons are made from metals because of their excellent heat conductivity.
-
Ductility is the ability of a material to be stretched into a wire. This property allows metals to be drawn into wires and, combined with their durability, makes them ideal for cable wires and soldering. Because metals can be drawn into wires, we say that metals are ductile.
-
Malleability is the property that allows substances to be beaten into flat sheets. Aluminum sheets, for instance, are used in the manufacturing of aircraft due to their lightweight and strength. Other metal sheets are used in the automobile industry and for making utensils. Therefore, metals are malleable.
-
Metals are sonorous, meaning they produce a deep or ringing sound when struck with a hard object.
-
While most metals naturally have a shiny appearance, they can also be polished to enhance their shine.
Chemical Properties of Metals
-
Reaction with water: Only highly reactive metals react with water, not all metals. For example, sodium reacts vigorously with water and oxygen, producing a large amount of heat. This is why sodium is stored in kerosene to prevent it from coming into contact with moisture or oxygen.
-
Reaction with acids: When metals react with acids, they produce hydrogen gas. For example, when zinc reacts with hydrochloric acid, it forms zinc chloride and hydrogen gas.
-
Reaction with bases: Not all metals react with bases, but when they do, they produce metal salts and hydrogen gas. For instance, when zinc reacts with strong sodium hydroxide, it forms sodium zincate and hydrogen gas.
-
Reaction with oxygen: Metals form metal oxides when they burn in the presence of oxygen. These metal oxides are basic in nature. For example, when magnesium is burned in oxygen, it forms magnesium oxide, which, when dissolved in water, becomes magnesium hydroxide.
Applications of Metals
Metals have a wide range of applications across various industries due to their versatile properties.
-
Construction and Infrastructure:
- Steel (Alloy of Iron): Used in buildings, bridges, railways, and other structures due to its high strength and durability.
- Aluminum: Commonly used in windows, doors, and roofing materials due to its lightweight and corrosion resistance.
- Copper: Used in electrical wiring and plumbing due to its excellent conductivity and corrosion resistance.
-
Electrical and Electronics:
- Copper and Aluminum: These metals are widely used in wires, cables, motors, and transformers because of their high electrical conductivity.
- Gold and Silver: Used in high-end electronic components and circuit boards due to their superior conductivity and corrosion resistance.
-
Transportation:
- Aluminum: Used in manufacturing aircraft, automobiles, and trains due to its lightweight and rust resistance.
- Steel: Used in the frames and bodies of cars, ships, and trains because of its strength and ability to withstand high pressures.
-
Medical Applications:
- Titanium: Used in medical implants, prosthetics, and surgical instruments due to its biocompatibility, corrosion resistance, and strength.
- Stainless Steel: Commonly used in medical equipment, surgical tools, and hospital structures.
-
Jewelry and Decoration:
- Gold, Silver, and Platinum: Precious metals used in jewelry, coins, and decorative items because of their rarity, beauty, and non-reactive nature.
-
Manufacturing and Machinery:
- Cast Iron and Steel: Used in the manufacture of machinery, tools, and industrial equipment due to their durability and wear resistance.
- Aluminum: Widely used in the manufacturing of products ranging from cans to complex machinery because of its machinability.
-
Aerospace Industry:
- Aluminum Alloys: Extensively used in aircraft and spacecraft construction because of their high strength-to-weight ratio and corrosion resistance.
- Titanium: Valued in aerospace for its strength and ability to withstand extreme temperatures.
-
Energy Production:
- Copper: Used in power generation and distribution systems, including generators, motors, and transformers.
- Steel: A key material in the production of wind turbines, oil pipelines, and nuclear reactors.
Introduction of Metal Oxides in Communication
Metal oxides are used as insulators, semiconductors, capacitors, and even as protective coatings. Their ability to control the movement of electrons and photons in devices makes them crucial in microelectronics, fiber optics, and radio frequency (RF) communication systems.
These materials have unique electrical, optical, and dielectric properties, making them essential in designing and manufacturing components for telecommunication, wireless networks, and optical communication systems.
Silicon (Si)
Silicon (Si) is a chemical element with atomic number 14, and it is one of the most abundant elements on Earth, second only to oxygen. Silicon is a metalloid, meaning it has properties of both metals and non-metals, and it is widely recognized for its semiconducting properties. In its pure form, silicon is a hard, brittle crystalline solid with a blue-grey metallic sheen.
Silicon plays a pivotal role in modern technology, particularly in electronics and communication. Due to its ability to conduct electricity under certain conditions but act as an insulator under others, silicon forms the foundation of the semiconductor industry.
Applications of Silicon
- Semiconductor Devices: Silicon is used to manufacture transistors, the building blocks of microprocessors, memory chips, and other integrated circuits.
- Solar Cells: Silicon is the primary material used in photovoltaic (solar) cells, which convert sunlight into electrical energy.
- Optical Communication: Silicon is used in photonic devices, such as optical modulators and detectors, in fiber optic communication systems.
- Glass Production: Silicon is a key component in producing glass and ceramics used in various industries.
Germanium (Ge)
Germanium (Ge) is a chemical element with atomic number 32. It belongs to the group of metalloids, similar to silicon. It is a lustrous, hard, grayish-white material with properties that make it useful in both metallic and non-metallic forms.
Germanium has regained importance in specialized areas due to its superior optical and electronic properties. It is a key material in high-speed electronics, fiber-optic systems, infrared optics, and photovoltaic devices.
Applications of Germanium
- Semiconductor Devices: Germanium is used in high-speed transistors, particularly heterojunction bipolar transistors (HBTs) and field-effect transistors (FETs), essential for high-frequency communication systems.
- Optical Fiber Communication: Germanium is added to glass (silica) in optical fibers to increase the refractive index, improving the efficiency and performance of fiber-optic cables for high-speed data transmission.
- Infrared Optics: Due to its transparency in the infrared spectrum, germanium is widely used in infrared cameras, thermal imaging devices, and infrared sensors used in military, medical, and industrial applications.
- Photodetectors and Solar Cells: Germanium is used in photodetectors, which convert light into electrical signals. These are crucial for communication technologies such as fiber optics. It is also used in high-efficiency multijunction solar cells.
Aluminum (Al)
Aluminum (Al) is a chemical element with atomic number 13, known for being lightweight, malleable, and corrosion-resistant. It is the most abundant metal in the Earth's crust, making up about 8% by weight, and is the second most widely used metal globally after iron. Aluminum is characterized by its silvery-white appearance and is highly valued in many industries due to its favorable properties.
Aluminum is found in nature primarily in the form of bauxite ore, from which it is extracted through the Hall-Héroult process. Its widespread use in industries such as aerospace, transportation, packaging, and electronics is due to its high strength-to-weight ratio, excellent conductivity, and resistance to corrosion.
Applications of Aluminum
- Aerospace and Automotive: Due to its lightweight and high strength, aluminum is used extensively in manufacturing aircraft, automobiles, and spacecraft, improving fuel efficiency and performance.
- Construction: Aluminum is used in buildings for windows, doors, roofing, and siding due to its corrosion resistance and ease of fabrication.
- Packaging: The metal’s non-toxic nature and resistance to corrosion make aluminum ideal for packaging food and beverages, in the form of cans, foils, and packaging films.
- Electrical Applications: Aluminum is used in power lines and electrical cables due to its excellent conductivity and lightweight properties, making it a cost-effective alternative to copper.
- Electronics and Communication: Aluminum is used in electronic components like heat sinks, cases, and conductors due to its thermal conductivity and lightweight nature.
Titanium (Ti)
Titanium (Ti) is a chemical element with atomic number 22. It is a transition metal known for its exceptional strength, lightweight, and resistance to corrosion. Titanium is silvery-gray in appearance and is commonly found in the Earth's crust in minerals such as rutile and ilmenite. Despite its relative abundance, titanium is expensive to extract and process due to its high melting point and complex refining process.
Titanium is highly valued in industries requiring materials that can withstand extreme environments, such as aerospace, medical, marine, and chemical processing. Its excellent mechanical properties and biocompatibility make it one of the most useful metals for specialized applications.
Applications of Titanium
- Aerospace Industry: Titanium’s high strength and low weight make it essential for aircraft frames, jet engines, and spacecraft. It is used in components that must withstand extreme forces and high temperatures.
- Medical Field: Titanium is widely used in medical implants, such as hip replacements, dental implants, and pacemaker housings, due to its biocompatibility and resistance to body fluids.
- Marine Industry: Titanium’s corrosion resistance makes it ideal for marine applications, such as shipbuilding, underwater equipment, and desalination plants, where exposure to seawater is constant.
- Chemical Processing: Titanium is used in chemical processing plants for equipment that must withstand corrosive environments, such as heat exchangers, tanks, and piping in producing chemicals like chlorine.
- Sports Equipment: Titanium’s lightweight yet strong properties make it a preferred material for high-end sports equipment, including golf clubs, tennis rackets, and bicycles.
Nickel (Ni)
Nickel (Ni) is a chemical element with atomic number 28, known for its silver-white, lustrous appearance, and excellent corrosion resistance and durability. Nickel is a transition metal and is commonly found in the Earth's crust, often associated with iron. It is primarily used in producing alloys due to its ability to enhance the properties of metals, such as strength, corrosion resistance, and toughness.
Nickel is highly valued in industries like aerospace, electronics, and energy due to its unique chemical and physical properties. It is also a key component in stainless steel, contributing to corrosion resistance and strength, making it critical for manufacturing and engineering applications.
Applications of Nickel
- Stainless Steel Production: Nickel is a key component in stainless steel, improving corrosion resistance and mechanical strength. Stainless steel is used in various applications, including kitchen appliances, construction materials, and medical instruments.
- Aerospace Industry: Nickel-based superalloys are used in jet engines, gas turbines, and other high-performance components that must withstand extreme temperatures and stresses.
- Electronics: Nickel is used in the production of batteries, particularly in rechargeable nickel-cadmium (NiCd) and nickel-metal hydride (NiMH) batteries, which power many consumer electronics.
- Corrosion-Resistant Coatings: Nickel is used as a protective coating for other metals, including electroplating, to prevent corrosion and wear. It is commonly applied to industrial machinery, automotive parts, and household appliances.
- Chemical Processing: Nickel is used in producing catalysts for chemical reactions, particularly in hydrogenation processes in the chemical and petrochemical industries.
- Coins and Currency: Many coins around the world are made with nickel or nickel alloys due to the metal's durability and resistance to wear.
Copper (Cu)
Copper (Cu) is a chemical element with atomic number 29, recognized for its reddish-brown color and excellent electrical and thermal conductivity. It is one of the earliest metals used by humans, dating back to ancient civilizations for tools, ornaments, and construction. Copper's unique combination of physical, chemical, and mechanical properties makes it a critical material in modern industries, particularly in electrical and electronic applications.
Copper is highly ductile and malleable, allowing it to be easily shaped into wires, sheets, and tubes. Its high resistance to corrosion and excellent conductivity make it ideal for electrical wiring, plumbing, and heat exchangers.
Applications of Copper
- Electrical Wiring and Electronics: Copper’s superior electrical conductivity makes it essential for electrical wiring in homes, buildings, and electronic devices. It is used in power transmission, motor windings, and circuit boards.
- Plumbing and Piping: Copper pipes are widely used for plumbing systems due to their corrosion resistance and ability to withstand both hot and cold water. It is also used in industrial applications for transporting gases and fluids.
- Heat Exchangers and Radiators: Copper's high thermal conductivity makes it ideal for radiators, heat exchangers, and air conditioning systems, where efficient heat transfer is required.
- Telecommunication and Data Transmission: Copper is used in communication cables, including coaxial cables, twisted pair cables, and fiber optics, where it facilitates the transmission of electrical signals and data.
- Alloys: Copper is alloyed with other metals to create materials like bronze (copper and tin) and brass (copper and zinc), used in various applications, from construction to musical instruments.
- Antimicrobial Surfaces: Copper's natural antimicrobial properties are used in healthcare settings, public spaces, and household products to reduce the spread of bacteria and viruses.
Silicon Dioxide ()
Silicon Dioxide (), also known as silica, is a chemical compound made up of silicon and oxygen, two of the most abundant elements in the Earth's crust. It occurs naturally in various forms, including quartz, sand, and many types of rocks. is a vital material in multiple industries due to its versatile physical and chemical properties. It is commonly found in crystalline, amorphous, or fused forms and is used in everything from construction materials to electronics.
Silicon dioxide is most commonly associated with glass production and the semiconductor industry, where it plays a crucial role as an insulator and protective layer in microelectronic devices.
Applications of Silicon Dioxide
-
Glass Manufacturing: Silicon dioxide is the primary ingredient in the production of glass. It is used in making windows, containers, and optical lenses due to its transparency and durability.
-
Semiconductor Industry: In electronics, is widely used in the production of integrated circuits (ICs) and other semiconductor devices. It serves as an insulating layer and a protective coating in microchips, enabling efficient functioning of electronic components.
-
Optical Fiber Communication: is used in optical fibers, where it enables the transmission of light over long distances with minimal signal loss. This is crucial for high-speed data communication in the telecommunications industry.
-
Construction Materials: Silicon dioxide is a major component of concrete, cement, and ceramics. In construction, it provides structural strength and thermal stability to materials used in buildings and infrastructure.
-
Abrasives and Polishing: Due to its hardness, is used as an abrasive in sandpaper, grinding tools, and polishing compounds for glass and metal surfaces.
-
Food and Pharmaceuticals: In powdered form, is used as an anti-caking agent in food products and supplements. It prevents clumping and improves the flow of powdered ingredients.
Lanthanum Oxide ()
Lanthanum Oxide () is a white, odorless, and hygroscopic solid that belongs to the class of rare earth metal oxides. Lanthanum (La) is a rare earth element, and its oxide is widely used in various advanced applications due to its unique properties, including a high refractive index, thermal stability, and catalytic behavior. It is commonly referred to as lanthana and is used in industries ranging from electronics and optics to catalysis and ceramics.
Applications of Lanthanum Oxide
-
Optical Glass and Lenses: Lanthanum oxide is added to glass formulations to improve the refractive index and reduce optical dispersion. It is commonly used in the production of high-quality lenses for cameras, microscopes, and telescopes.
-
Catalysis: is used as a catalyst or catalyst support in various chemical reactions, particularly in refining and petrochemical industries. It enhances the efficiency of processes like petroleum cracking and the production of synthetic fuels.
-
Electronics and Communication: Lanthanum oxide is used in electronic materials, including dielectric and insulating layers in capacitors and semiconductors. It plays a critical role in enhancing the performance of communication systems, especially in high-frequency applications.
-
Ceramics and Batteries: Lanthanum oxide is used in ceramic formulations to improve mechanical strength and thermal stability. It is also employed in the production of solid oxide fuel cells (SOFCs) and certain types of rechargeable batteries.
-
**Phosphors:**In combination with other rare earth elements, is used in phosphors for lighting and display technologies, such as fluorescent lamps and LED screens.
Zirconium Dioxide ()
Zirconium dioxide, commonly known as zirconia (), is a white crystalline oxide of zirconium. It has gained significant attention in various fields due to its unique properties. Zirconia can exist in several crystal forms, including monoclinic, tetragonal, and cubic, depending on the temperature and other conditions.
Zirconium dioxide is essential in both advanced and traditional applications due to its strength, thermal stability, and electrical properties. Its versatility makes it a valuable material in fields ranging from medicine to aerospace engineering.
Applications of Zirconium Dioxide
-
Ceramics and Refractories: Zirconia is used in dental crowns, bridges, and implants due to its aesthetic appeal, strength, and biocompatibility. It is utilized in various industrial applications, such as cutting tools, wear-resistant components, and thermal barrier coatings.
-
Electrolytes: Zirconia is used in solid oxide fuel cells (SOFCs) and oxygen sensors due to its ionic conductivity, allowing for efficient ionic transport at high temperatures.
-
Catalysis: Zirconia is employed as a catalyst support in various chemical reactions, improving reaction rates and selectivity.
-
Optics: Due to its high refractive index and low dispersion, zirconia is used in optical applications, including lenses and coatings.
Display Devices
Display devices are electronic components that visually present information in a way that can be easily interpreted by the human eye. They convert electrical signals into visual signals, which can take the form of text, images, or video.
Liquid Crystal-Based Displays (LCD)
Liquid crystal displays (LCDs) are among the most widely used display technologies today, owing to their energy efficiency, slim form factor, and ability to produce high-quality images. LCDs are primarily used in devices such as televisions, computer monitors, smartphones, and digital watches.
LCDs operate using liquid crystals, which are substances that exhibit properties between those of liquids and solid crystals. These liquid crystals do not emit light directly; instead, they manipulate light passing through them to display images. This manipulation is controlled through an external light source, typically a backlight, and an electric field.
In LCD displays, millions of pixels made of liquid crystals are arranged in a rectangular grid. Each pixel contains red, green, and blue (RGB) sub-pixels that can be turned on or off. When all sub-pixels are turned off, the pixel appears black, and when all are turned on at 100%, it appears white.
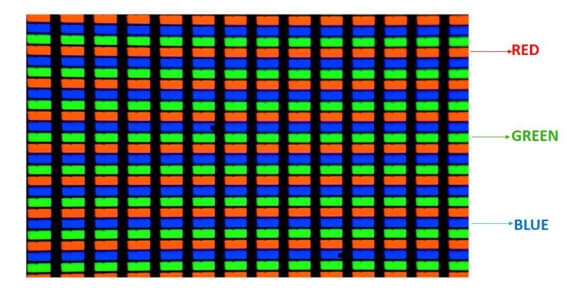
LCDs are a combination of two states of matter: solid and liquid. The solid part is crystal, and together with the liquid, it forms the visible image. An LCD consists of two layers: two polarized panels (filters) and electrodes.
An LCD screen works by blocking light rather than emitting it. There are two types of pixel grids in LCDs:
- Active Matrix Grid: This is a newer technology. Smartphones with LCD displays use this technology.
- Passive Matrix Grid: This is an older technology, which was used in some earlier devices.
Different Types of LCDs
There are mainly four types of LCDs available:
-
Twisted Nematic (TN):
These are affordable but have fast response times. However, TN screens have low contrast ratios, limited viewing angles, and poor color accuracy. Despite these drawbacks, TN LCDs are widely manufactured and used in many types of displays across various industries. -
In-Plane Switching (IPS) Displays:
Compared to TN LCDs, IPS displays have significantly higher contrast ratios, wider viewing angles, and better color accuracy. IPS panels provide superior picture quality, vibrant color reproduction, and better image consistency compared to TN panels. -
Vertical Alignment (VA) Panels:
VA panels are considered an intermediate technology between TN and IPS displays. They offer a good compromise, with better color reproduction and viewing angles compared to TN displays. VA panels are known for their deeper blacks and higher contrast than IPS panels but may not match the response time of TN panels. -
Advanced Fringe Field Switching (AFFS):
AFFS is a top performer in color reproduction, surpassing even IPS displays. AFFS panels are known for producing high-quality images and are commonly used in high-tech applications, such as in airplane cockpits.
How LCDs Work?
LCDs operate based on the principle of switching pixels on and off to display specific colors using liquid crystals. When an electric current is applied to the liquid crystal molecules, they untwist, changing the angle of the top polarizing filter and altering the light passing through the polarized glass.
As a result, in certain areas of the LCD, light is blocked, causing the area to appear dark. Unlike other display technologies, LCDs do not emit light but instead work by blocking it. A reflective mirror is placed behind the LCD during construction, and indium-tin-oxide is used to create the electrode plane, which is positioned on top of the device. A polarized glass with a polarizing film is placed on the bottom.
The entire area of the LCD must be covered by a common electrode, with the liquid crystal material layered above it. On top of this comes another polarizing film, while on the bottom, a second piece of glass with an electrode is arranged in a rectangular form. These two pieces of glass are aligned at right angles to each other. When no current is applied, light passes through the LCD, reflects off the mirror, and bounces back.
A screen composed of pixels colored red, green, and blue is placed in front of the light. The liquid crystals control filters to either display a particular color or keep the pixel black. This is why LCD monitors and TVs consume significantly less power compared to CRT monitors or televisions.
Advantages of LCDs
- The main advantage of LCDs is that they are low-cost and energy-efficient, consuming very little power.
- LCDs are thinner, lighter, and more flexible compared to older technologies.
- LCDs provide excellent contrast, brightness, and resolution, resulting in crystal-clear picture quality.
- Radiation emitted by LCD monitors is much lower than that from CRT monitors.
- LCDs are compatible with CMOS integrated circuits, making manufacturing easier.
- They offer perfect sharpness at their native resolution.
- LCDs have zero geometric distortion at their native resolution.
- LCDs offer greater portability compared to earlier screen technologies.
Disadvantages of LCDs
- LCDs require an external light source to illuminate the pixels. If the light source fails, the display will not show any image.
- LCDs are less reliable than some other display types.
- Image visibility depends on light intensity.
- The aspect ratio and resolution of an LCD are fixed.
- LCDs have an irregular intensity scale and can produce fewer than 256 discrete intensity levels.
- Color saturation is reduced at low intensity levels due to poor black-level performance.
- LCDs have limited viewing angles, which affect brightness and cause color distortion when viewed from an angle.
Light Emitting Diode (LED)
A light-emitting diode (LED) is a semiconductor device that emits light when an electric current flows through it. When current passes through an LED, electrons recombine with holes, emitting light in the process. LEDs allow current to flow in the forward direction and block current in the reverse direction.
Light-emitting diodes are heavily doped p-n junctions. Depending on the semiconductor material used and the level of doping, an LED will emit colored light at a particular spectral wavelength when forward biased. An LED is encapsulated with a transparent cover so that the emitted light can pass through.
LED Symbol
The LED symbol is the standard symbol for a diode, with the addition of two small arrows indicating the emission of light.
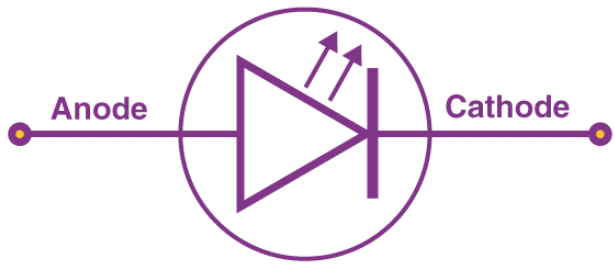
Simple LED Circuit
The figure below shows a simple LED circuit. The circuit consists of an LED, a voltage supply, and a resistor to regulate the current and voltage.
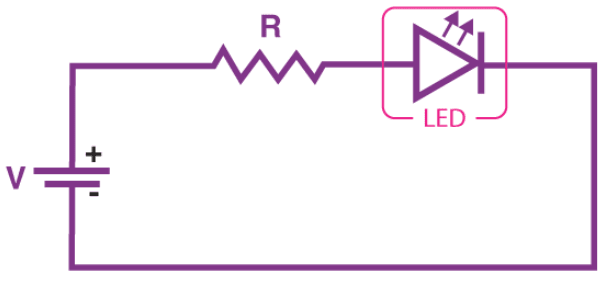
How Does an LED Work?
When the diode is forward biased, minority electrons move from the p-region to the n-region, while minority holes move from the n-region to the p-region. At the junction boundary, the concentration of minority carriers increases. The excess minority carriers recombine with majority charge carriers at the junction.
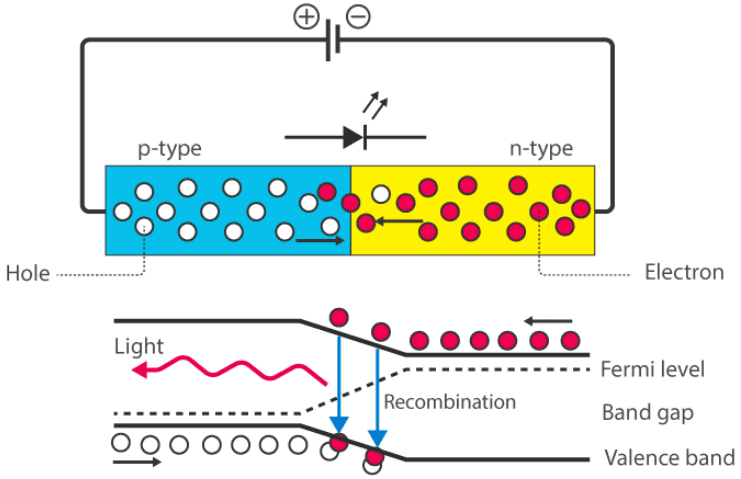
Energy is released in the form of photons during recombination. In standard diodes, this energy is released as heat. However, in light-emitting diodes, the energy is released in the form of light photons. This phenomenon is called electroluminescence, an optical and electrical process where a material emits light in response to an electric current passing through it. As the forward voltage increases, the intensity of the emitted light increases until it reaches a maximum.
The color of an LED is determined by the material used in the semiconductor. The two primary materials used in LEDs are aluminum gallium indium phosphide alloys and indium gallium nitride alloys. Aluminum alloys are used to produce red, orange, and yellow light, while indium alloys are used to produce green, blue, and white light. Slight changes in the composition of these alloys can change the color of the emitted light.
Advantages of LED Displays
- Energy Efficiency: LEDs consume less power compared to older technologies like fluorescent or incandescent bulbs, making them highly energy-efficient.
- Brightness and Contrast: LED displays offer high brightness and contrast ratios, making them suitable for both indoor and outdoor environments, even in areas with high ambient light.
- Longevity: LEDs have a long operational lifespan, often lasting tens of thousands of hours, making them more durable and cost-effective in the long run.
- Fast Response Time: LEDs have faster response times compared to other display technologies, reducing motion blur and improving image clarity, especially for video content.
- Flexibility: OLEDs, a type of LED, can be made on flexible substrates, allowing for curved or foldable displays, particularly useful in smartphones and other portable devices.
Disadvantages of LED Displays
- Cost: High-quality LED and OLED displays can be more expensive to produce than other display technologies like LCDs.
- Image Retention (Burn-In) in OLEDs: OLED displays can suffer from image retention, where ghost images remain visible after prolonged display of static images.
- Viewing Angles: Some LED displays (like TN-based LED-backlit displays) have limited viewing angles, though this has improved with technologies like IPS in LED-backlit LCDs.
Cathode Ray Tube (CRT)
CRT stands for Cathode Ray Tube, a technology used in traditional computer monitors and televisions. The image on a CRT display is created by firing electrons from the back of the tube at a phosphorus-coated screen. When the electrons hit the phosphorus, it lights up, and the image is projected on the screen. The colors you see are produced by a combination of red, blue, and green light.
Components of CRT
-
Electron Gun: The electron gun consists of a series of elements, primarily a heating filament (heater) and a cathode. It creates a source of electrons, which are focused into a narrow beam directed at the face of the CRT.
-
Control Electrode: Used to turn the electron beam on and off.
-
Focusing System: Ensures a clear picture by focusing the electron beam into a narrow stream.
-
Deflection Yoke: Controls the direction of the electron beam. It creates an electric or magnetic field that bends the electron beam as it passes through. In a conventional CRT, the yoke is connected to a sweep or scan generator.
-
Phosphor-Coated Screen: The inner front surface of the CRT is coated with phosphors, which glow when hit by high-energy electron beams. Phosphorescence refers to the light emitted by the phosphor after exposure to the electron beam.
Types of CRT Displays
-
Monochrome CRT: Uses a single electron gun and a single layer of phosphor coating, emitting one color (usually green, white, or amber).
- Applications: Early televisions, computer monitors, and oscilloscopes.
-
Color CRT: Uses three electron guns and three layers of phosphor dots (red, green, and blue) to create full-color images.
- Applications: Color televisions, computer monitors, and arcade game displays.
Working of Cathode Ray Tube
In a CRT, electrons are accelerated using an electric field from one end of the tube to the other. When the electrons reach the far end, they lose energy, which is converted into heat and sometimes a small amount of X-rays.
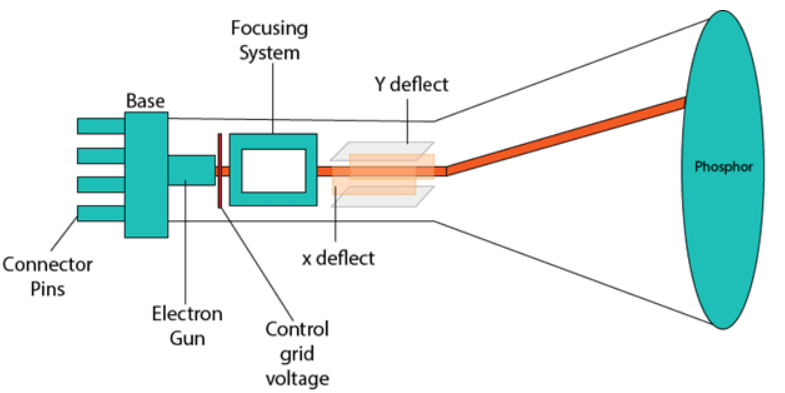
The electron beams in the display (interlaced or non-interlaced) travel across the screen, striking phosphorus dots inside the glass tube, causing them to light up and project the image onto the display. There are three primary electron beams—green, red, and blue. Speakers or magnetic devices placed near a CRT display can cause interference due to the magnetic charges that influence electron direction. This issue doesn't affect LCDs and flat screens.
Advantages of CRT Displays
- Superior Color Accuracy and Contrast: CRTs are known for excellent color reproduction and deep contrast ratios, making them ideal for professional use in graphic design, video editing, and broadcast monitoring.
- Wide Viewing Angles: CRTs offer consistent image quality across wide viewing angles, unlike early LCDs.
- Fast Response Time: CRTs refresh at the speed of the electron beam, eliminating motion blur and ghosting, making them ideal for fast-moving content like video games and action scenes.
- High Resolution and Flexibility: CRTs can display multiple resolutions natively and handle various aspect ratios without losing image quality.
Disadvantages of CRT Displays
- Bulk and Weight: CRTs are bulky and heavy due to their deep tube design. Larger CRTs (over 20 inches) are difficult to transport and occupy significant space.
- Power Consumption: CRTs are not energy-efficient compared to modern flat-panel displays. They consume more power and generate more heat.
- Flicker at Lower Refresh Rates: At refresh rates below 60 Hz, CRTs can flicker, causing eye strain during extended use.
- Magnetic Sensitivity: CRTs are sensitive to magnetic fields, which can distort the image. Devices like speakers or magnets placed near a CRT can interfere with its operation.
Alumina-Silicate Glass-Based Displays
Alumina-silicate glass is a type of glass commonly used in the protective layers of modern display devices, such as smartphones, tablets, and some high-end monitors. While it doesn't directly produce images like CRTs or LEDs, alumina-silicate glass plays a critical role in making displays more durable, scratch-resistant, and shatterproof. This glass is often chemically strengthened to improve its performance in devices where strength and durability are important.
Alumina-silicate glass is made from a mixture of alumina and silica , along with other elements like sodium (Na), potassium (K), and calcium (Ca). It differs from traditional soda-lime glass (used in windows and bottles) by having aluminum oxide (alumina), which increases its toughness and resistance to mechanical damage.
Key properties of alumina-silicate glass:
- High strength: Alumina makes the glass significantly stronger than regular glass.
- Lightweight: Despite its strength, it remains lightweight, making it ideal for portable devices.
- High scratch resistance: The glass is highly resistant to scratching, an essential quality for devices that are handled frequently.
- Resistance to heat and shock: It can withstand high temperatures and rapid temperature changes without cracking.
How It Works in a Display?
- Protection Layer: Alumina-silicate glass acts as the first line of defense, absorbing shocks, impacts, and scratches that would otherwise damage the sensitive electronic components beneath, such as LCD or OLED panels.
- Touchscreen Compatibility: The glass must remain thin and transparent to allow touch sensitivity, especially in capacitive touchscreens. Alumina-silicate glass is used because of its optical clarity and responsiveness to touch, making it ideal for smartphone and tablet displays.
- Optical Clarity: Alumina-silicate glass has excellent light transmission properties, which means it does not degrade the image quality of the underlying display, ensuring sharp, vibrant images.
Advantages of Alumina-Silicate Glass in Displays
- Durability: Alumina-silicate glass is highly resistant to scratches, drops, and impacts, making it ideal for everyday use in smartphones, tablets, and other touch-sensitive devices.
- Thin and Lightweight: Despite its strength, it is thin and lightweight, allowing for sleek and lightweight device designs without compromising durability.
- Heat Resistance: It can withstand high temperatures, making it less likely to crack under rapid temperature changes or direct exposure to heat.
- Improved Touch Sensitivity: Alumina-silicate glass allows for high sensitivity in touchscreens, making it an excellent choice for devices that rely on precise touch input.
Disadvantages of Alumina-Silicate Glass:
- Expensive Manufacturing: The chemical strengthening process can be costly, increasing the overall price of devices using alumina-silicate glass, especially high-end devices like flagship smartphones.
- Still Breakable: While it is more durable than regular glass, it is not indestructible. A sufficiently strong impact can still crack or shatter the glass.
Touchscreen Display
A touchscreen is an electronic visual display that can detect the presence and location of a touch (usually by a finger or hand) within the display area. Touchscreens are a useful alternative to a mouse and keyboard for navigating a graphical user interface (GUI). Some devices also use touch displays equipped with a grid of infrared beams that detect a finger, reducing the requirement for touch-sensitive input.
Touchscreens first appeared in the 1960s. E.A. Johnson invented the first touch-driven screen in 1965. This technology received little attention until the 1970s, when a research team at the University of Kentucky in the United States accidentally produced a resistive touchscreen. In the 1980s, the University of Toronto developed the first multi-touch display. The first touchscreen cell phones were introduced in the 1980s.
Components of a Touchscreen
- Touch Sensor: The structure of touch sensors depends on the type of touchscreen being used. Its function is to detect any valid touch, typically from a finger or hand.
- Controller: A controller is a small PC card that communicates the location of the touch to the device's software.
- Software Drivers: Software drivers allow the operating system to interface with the touchscreen. These drivers are typically developed using the C programming language.
Working of Touchscreen Technology
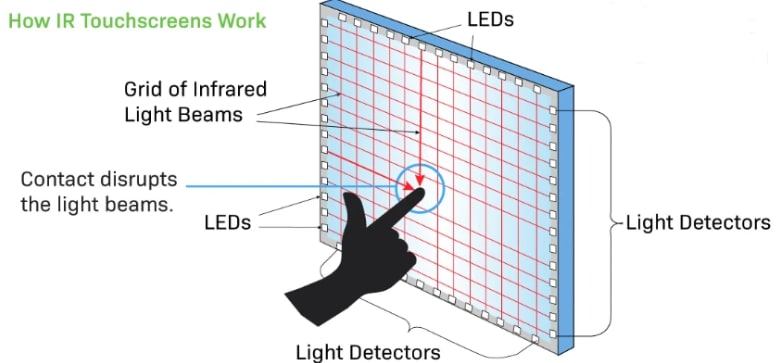
Touchscreen technology works on several principles, each used to detect and respond to touch inputs. Capacitive touchscreens, one of the most common types, work by measuring changes in capacitance when a conductive object, such as a finger, touches the screen, disrupting an electrostatic field. Resistive touchscreens work by pressing two flexible layers together, completing a circuit and detecting touch based on changes in electrical current flow. Surface Acoustic Wave (SAW) touchscreens emit ultrasonic waves over the screen's surface, and touchpoints are identified by disruptions in the wave pattern. Infrared touchscreens use infrared light beams across the screen, detecting touch points based on interruptions in the beams. These technologies collectively enable intuitive interaction with electronic devices, revolutionizing user interfaces across a wide range of applications.
Types of Touchscreens
1. Resistive Touchscreen: This is the simplest and most commonly used touchscreen. Resistive touchscreens require significant pressure to register a touch, hence the name. They consist of two layers: a resistive outer layer and a conductive inner layer. These layers are separated by tiny dots called spacers. When the screen is touched, the layers are pressed together, changing the electrical current. The software detects this change and performs the corresponding function. Advantages include reliability and durability, but drawbacks include difficulty reading the screen in bright light and the inability to recognize multiple touch points simultaneously.
2. Capacitive Touchscreen: Capacitive screens are made of indium tin oxide or copper, which store electrical charges in very thin wires. The capacitive screen detects changes in electric current when it comes into contact with anything that holds a charge, such as human skin. There are two types of capacitive screens:
- Projected Capacitive: Uses a grid of sensor chips.
- Surface Capacitive: Uses sensors at the screen corners and a paper-thin film over the surface. When touched, a small amount of electrical charge is transferred to the finger, creating a complete circuit and causing a voltage drop in a specific location. The software analyzes this voltage drop and performs the corresponding action.
3. Infrared Touchscreen: Infrared touchscreens shine infrared light in a grid pattern across the screen. When someone touches the screen, some of the infrared beams are interrupted, and the microchip detects the location of the interruption to perform the desired action.
4. Surface Acoustic Wave Technology: This technology uses ultrasonic sound waves to detect touch. The screen emits ultrasonic waves at its edges, which reflect across the surface. When a finger touches the screen, the sound waves are disrupted, and the microchip controller detects the location of the touch by analyzing the disruption.
Advantages of Touchscreens
- Reduces errors during navigation.
- Provides easy and fast access to files.
- Eliminates the need for additional input devices, like a keyboard or mouse, saving energy.
- Improves security.
Disadvantages of Touchscreens
- They are not suitable for storing large amounts of data.
- Installing multiple touchscreen devices can be costly and not always cost-effective.
Your paragraph is already well-written, but I’ve made some small grammatical and stylistic improvements to ensure clarity and consistency. Here is the revised version, along with explanations:
Disposal of Harmful Chemicals Used in ICT
Information and Communication Technology (ICT) devices, such as computers, smartphones, televisions, and other electronics, contain various hazardous chemicals and materials that pose environmental and health risks when improperly disposed of. Some of the most concerning chemicals include mercury (Hg), lead (Pb), cadmium (Cd), and flame retardants. These substances can contaminate soil, air, and water, leading to long-term ecological damage and health problems for humans and wildlife.
Proper disposal and recycling methods are critical to minimizing the environmental impact of these chemicals.
Disposal of Harmful Chemicals in ICT
-
E-Waste Recycling: The safest and most environmentally responsible way to dispose of ICT devices containing hazardous chemicals is through certified e-waste recycling programs. These programs ensure that harmful substances are safely extracted, recycled, or disposed of without causing environmental harm.
Take-back programs offered by manufacturers, retailers, or local governments allow consumers to return old electronics for safe disposal and recycling. -
Extended Producer Responsibility (EPR): Under EPR, manufacturers are responsible for the disposal of their products at the end of their life cycle. This ensures that companies participate in the recycling and safe disposal of harmful materials like lead, mercury, and flame retardants.
-
Government Regulations: Many countries have laws, such as the Restriction of Hazardous Substances (RoHS) directive in the EU, which limit the use of hazardous substances like lead, mercury, cadmium, and certain flame retardants in electronic devices. Other policies, like WEEE (Waste Electrical and Electronic Equipment) directives, promote the recycling and proper disposal of e-waste.
-
Consumer Responsibility: Consumers can reduce the environmental impact of harmful chemicals by properly disposing of their electronics at designated e-waste recycling centers and avoiding illegal dumping or burning of electronics.
Mercury (Hg)
Mercury is used in small amounts in various electronic components, including:
- LCD displays (as a component of backlighting)
- Thermostats
- Batteries
- Switches and relays
Environmental and Health Risks
- Mercury is highly toxic and can damage the nervous system, kidneys, and lungs. It also accumulates in the food chain, particularly in fish, leading to bioaccumulation and biomagnification.
- Once mercury enters the environment, it can convert to methylmercury, a highly toxic compound that is particularly dangerous to developing fetuses and young children.
Disposal Methods
- Recycling: Mercury-containing products should be collected separately and sent to specialized recycling centers. Mercury can be extracted and reused safely in new products or stored for long-term containment.
- Proper Collection: Many countries and regions have established programs for collecting mercury-containing electronics, such as LCD monitors and compact fluorescent bulbs (CFLs), to prevent mercury from entering landfills.
- Incineration Prohibition: Mercury-containing electronics should never be incinerated, as burning them can release toxic mercury vapor into the atmosphere.
Lead (Pb)
Lead has been widely used in:
- Soldering components on circuit boards
- CRT (Cathode Ray Tube) displays in older TVs and monitors
- Batteries
- Cables and connectors
Environmental and Health Risks
- Lead exposure can cause neurological disorders, kidney damage, and developmental issues, especially in children. Chronic exposure to lead can result in reduced cognitive function, anemia, and immune system suppression.
- Lead can persist in the environment for long periods, contaminating soil and water.
Disposal Methods
- E-Waste Recycling: Lead-containing electronics should be processed at e-waste recycling centers where lead can be recovered and properly disposed of. Circuit boards and CRT monitors should never be thrown in landfills.
- CRT Recycling: CRT recycling involves separating the lead from the glass, allowing both materials to be reused. Specialized recycling plants process CRT monitors to ensure lead is safely extracted.
- Batteries: Lead-acid batteries should be sent to certified battery recycling programs. These batteries can be recycled, with lead being recovered for reuse in new batteries.
Cadmium (Cd)
Cadmium is found in:
- Rechargeable batteries (Nickel-Cadmium or NiCd batteries)
- Semiconductors and certain electrical contacts
- Plastics (used as a stabilizer and colorant)
Environmental and Health Risks
- Cadmium is a known carcinogen and can cause kidney, lung, and bone damage. It is also harmful to aquatic life and can bioaccumulate in the food chain.
- Cadmium remains in the environment for long periods, contaminating soil and water.
Disposal Methods
- Battery Recycling: NiCd batteries should be disposed of through proper battery recycling programs, where cadmium can be safely extracted and reused or stored in a secure facility.
- E-Waste Collection: Electronic devices containing cadmium should be sent to specialized e-waste recycling centers to prevent cadmium from being released into the environment.
- Landfill Prohibition: Cadmium-containing waste should never be dumped in landfills or incinerated, as this can lead to cadmium leaching into groundwater or being released into the air as toxic fumes.
Flame Retardants
Flame retardants are chemicals added to electronics to reduce their flammability. These substances are used in:
- Plastic casings of computers, televisions, and smartphones
- Printed circuit boards
- Cables and connectors
Common types of flame retardants include polybrominated diphenyl ethers (PBDEs), tetrabromobisphenol A (TBBPA), and hexabromocyclododecane (HBCD).
Environmental and Health Risks
- Flame retardants can disrupt the endocrine system and have been linked to developmental and reproductive issues, as well as cancer.
- These chemicals are persistent in the environment, accumulating in wildlife and humans, especially through dust particles in homes and offices.
- They also leach into soil and water, harming aquatic life.
Disposal Methods
- Specialized Recycling: Flame retardants in electronics can be difficult to recycle, but specialized e-waste recyclers are equipped to handle these materials safely.
- Separation in E-Waste Plants: In recycling facilities, flame retardant-containing plastics can be separated from other materials, and flame retardants can be safely disposed of or treated to minimize environmental harm.
- Avoid Open Burning: Open burning or incineration of flame retardant materials should be strictly avoided as it can release highly toxic compounds like dioxins and furans into the atmosphere.