Introduction
Electrochemistry is a branch of chemistry that deals with the interconversion of electrical energy and chemical energy, and vice versa.
For example:
- In a battery, chemical energy is converted to electrical energy.
- In electroplating or electrolysis, electrical energy is converted to chemical energy.
Electric current is a flow of electrons. Substances that allow electric current to pass through them are known as conductors. Examples include metals, graphite, fused salts, and aqueous solutions of acids, bases, and salts.
In contrast, an insulator or non-conductor is a substance that does not allow electric current to pass through it. Examples include wood and plastic.
Specific, Equivalent and Molar Conductivity of Electrolytic Solutions
Electric current is a flow of electrons. Substances that allow electric current to pass through them are known as conductors. Examples include metals, graphite, fused salts, and aqueous solutions of acids, bases, and salts.
Conductors are of two types:
-
Metallic Conductors: These substances conduct electricity through electrons. Examples include all metals, graphite, sodium (Na), potassium (K), alkaline earth metals, copper (Cu), silver (Ag), gold (Au), and other transition metals.
-
Electrolytic Conductors: These substances, in aqueous solution or in a fused state, liberate ions and conduct electricity through these ions, resulting in chemical decomposition. Examples include solutions of acids, bases, and salts.
Conductance:
Conductance is the reciprocal of resistance, defined as .
- For metallic conductors, resistance is a characteristic property. In contrast, electrolytes are characterized by conductance rather than resistance.
- The resistance of a conductor (metallic) is directly proportional to its length and inversely proportional to its cross-sectional area (Ohm’s Law).
where is the resistance in ohms, is the specific resistance, is the length in cm, and is the cross-sectional area in .
Thus, when and , then .
The specific resistance is defined as the resistance of a 1-centimeter cube.
Specific Conductivity
Specific conductivity () is the reciprocal of the specific resistance of an electrolytic solution.
Thus, specific conductivity represents the conductance of of a solution.
Units:
Where .
Equivalent Conductivity
Equivalent conductivity () is the conductance of all the ions liberated by 1 gram equivalent of the electrolyte at dilution. If 1 gram equivalent of electrolyte is present in , then:
Where is the volume that contains 1 gram equivalent of the electrolyte.
If the normality of the electrolytic solution is , then:
Thus:
Units:
Molar Conductivity
Molar conductivity () is defined as the conductance of all the ions produced by 1 mole of an electrolyte at dilution.
If 1 mole of electrolyte is present in of solution, then:
Where is the molar concentration in .
Then:
Units:
Reference Electrodes: Calomel Electrode and Ag-AgCl Electrode
In potentiometry, we measure the difference between the potential of two electrodes. The potential of one electrode—the working or indicator electrode—responds to the analyte’s activity, while the other electrode—the counter or reference electrode—has a known, fixed potential. By convention, the reference electrode is the anode; thus, the shorthand notation for a potentiometric electrochemical cell is:
reference electrode || indicator electrode
The cell potential is given by:
The ideal reference electrode provides a stable, known potential so that we can attribute any change in to the analyte’s effect on the indicator electrode’s potential. In addition, the reference electrode should be easy to construct and use.
Calomel Electrodes
A calomel electrode, also known as a mercury/mercurous chloride electrode, is a common reference electrode used in electrochemical measurements. Its main function is to provide a stable reference potential for comparison and calibration of potentials in the solution being measured.
A calomel electrode typically consists of a mercury/mercurous chloride (Hg/HgCl) electrode in a saturated potassium chloride (KCl) solution, where the mercury/mercurous chloride electrode measures the potential, and the saturated KCl solution provides a stabilized electrode response.
The principle of operation of a calomel electrode is based on the chemical reaction between the mercury/mercurous chloride electrode and a saturated potassium chloride solution. The mercury/mercurous chloride electrode is a half-cell coated with mercurous chloride (HgCl). When this electrode is immersed in a saturated KCl solution, a reaction between chloride ions and the mercury electrode occurs, creating an equilibrium of mercury and chloride ions. During this process, the concentration of silver ions remains constant, resulting in a stable potential.
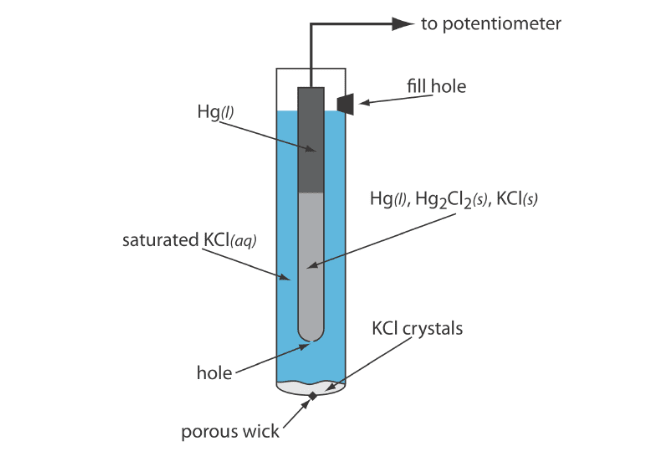
The electrode reaction of a calomel electrode can be expressed as:
For which the potential is:
The potential of a calomel electrode, therefore, depends on the activity of in equilibrium with and .
In a saturated calomel electrode (SCE), the concentration of is determined by the solubility of KCl. The electrode consists of an inner tube packed with a paste of Hg, HgCl, and KCl, situated within a second tube that contains a saturated solution of KCl. A small hole connects the two tubes, and a porous wick serves as a salt bridge to the solution in which the SCE is immersed. A stopper in the outer tube provides an opening for adding additional saturated KCl. The shorthand notation for this cell is:
Because the concentration of is fixed by the solubility of KCl, the potential of an SCE remains constant even if some of the inner solution is lost to evaporation. A significant disadvantage of the SCE is that the solubility of KCl is sensitive to changes in temperature. At higher temperatures, the solubility of KCl increases, and the electrode’s potential decreases.
Advantages of Calomel Electrodes
- High Stability: Calomel electrodes exhibit good stability under certain conditions, providing reliable potential measurements.
- Good Reversibility: The reaction reversibility of the calomel electrode is excellent, allowing for rapid response and recovery.
- Wide Range of Applications: Calomel electrodes are suitable for measuring pH in various solutions and have a wide range of applications.
- High Accuracy: The calomel electrode can provide high measurement accuracy under appropriate conditions.
- Ease of Use: Calomel electrodes are simple to use and do not require complex maintenance or operating procedures.
Silver/Silver Chloride Electrodes
Another common reference electrode is the silver/silver chloride electrode, which is based on the reduction of AgCl to Ag:
As with the calomel electrode, the activity of determines the potential of the Ag/AgCl electrode. Thus:
When prepared using a saturated solution of KCl, the electrode's potential is +0.197 V at 25°C. Another common Ag/AgCl electrode uses a solution of 3.5 M KCl and has a potential of +0.205 V at 25°C. As expected, the potential of an Ag/AgCl electrode using a saturated solution of KCl is more sensitive to changes in temperature than an electrode that uses an unsaturated solution of KCl.
A typical Ag/AgCl electrode consists of a silver wire, the end of which is coated with a thin film of AgCl, immersed in a solution containing the desired concentration of KCl. A porous plug serves as the salt bridge. The shorthand notation for this electrode is:
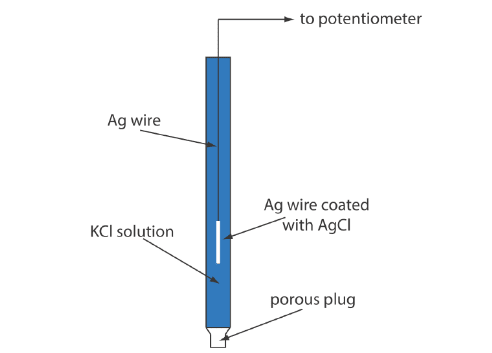
Advantages of Silver/Silver Chloride Electrodes
- Stability and Reproducibility: The Ag/AgCl electrode provides stable and reproducible potential readings, which are critical for accurate electrochemical measurements.
- Non-Toxic: Compared to other reference electrodes like the calomel electrode, the Ag/AgCl electrode is less toxic, as it does not contain mercury.
- Easy Preparation: It can be easily prepared by coating a silver wire with silver chloride, making it convenient for laboratory use.
- Wide Availability: The materials required (silver and silver chloride) are readily available and inexpensive, contributing to its widespread use.
- Versatility: The Ag/AgCl electrode can be used in various solutions, including those of varying ionic strengths and pH levels, enhancing its versatility in different applications.
Ion-Selective Electrode: Glass Electrode
- An ion-selective electrode (ISE) is an analytical technique used to determine the activity of ions in an aqueous solution by measuring the electrical potential.
- Specific ions dissolved in a solution create an electrical potential, which can be measured by a voltmeter or pH meter.
- The strength of this charge is directly proportional to the concentration of the selected ion.
- An ISE consists of a thin membrane that selectively allows only specific ions to diffuse through.
- By measuring the electric potential generated across a membrane by selected ions and comparing it with a reference electrode, the net charge can be determined.
Glass Electrode
The glass electrode is a type of ion-selective electrode that is widely used for measuring the pH of solutions. It functions based on the selective permeability of glass to hydrogen ions () and is crucial in various analytical chemistry applications.
Working Principle
The glass electrode operates on the principle of ion selectivity, particularly for hydrogen ions. It consists of a thin glass membrane that allows the diffusion of H⁺ ions while excluding other ions. When the electrode is immersed in a solution, a potential difference develops across the glass membrane due to the difference in concentration between the internal reference solution and the external solution.
Construction
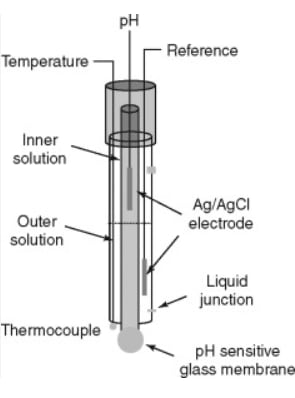
- Glass Membrane: The key component of the glass electrode is the specialized glass membrane, which is sensitive to H⁺ ions. The glass is typically made from a silicate material containing alkali metal ions.
- Internal Reference Solution: The interior of the electrode contains a stable reference solution, usually a buffered solution of known pH, in contact with a reference electrode (commonly an Ag/AgCl electrode).
- Outer Junction: The electrode typically has an outer junction that allows for electrical contact with the test solution.
- Protective Body: The glass electrode is often encased in a protective body made of plastic or glass to prevent damage.
Determination of pH of Solutions Using a Glass Electrode
pH is a measure of the hydrogen ion concentration in a solution and describes the degree of acidity or alkalinity (basicity) of a solution. pH measurement is most often used industrially in aqueous (water-based) applications. The scale for most common applications ranges from 0 to 14. A pH of 0 represents a concentration of 1 (or 100) mole/liter of hydrogen ions, while a pH of 14 represents 0.0000000000001 (10⁻¹⁴) moles/liter of hydrogen ions.
The pH value of a solution is measured using a pH electrode, which consists essentially of a pair of electrodes: a measurement electrode and a reference electrode, both submerged in the solution of unknown pH. These two electrodes essentially form two half-cells. Although the potential developed in the reference cell is constant, the potential of the measurement cell depends on the concentration of hydrogen ions in the solution and is governed by the Nernst equation:
Where:
- = electromotive force (e.m.f) of the half-cell
- = e.m.f of the half-cell under standard conditions
- = gas constant (8.314 J/K·mol)
- = absolute temperature (K)
- = valence of the ion
- = Faraday's constant (96493 C)
- = activity coefficient (0 ≤ a ≤ 1); for a very dilute solution,
- = molar concentration of ions
Advantages
- High Sensitivity: The glass electrode is highly sensitive to changes in hydrogen ion concentration, allowing for precise pH measurements.
- Wide Range of Measurement: It can measure pH across a broad range (typically pH 0 to pH 14).
- Fast Response Time: The glass electrode exhibits quick response times to changes in pH, making it suitable for dynamic measurements.
- Reusable: With proper care, glass electrodes can be reused multiple times, reducing costs in the long run.
- Robustness: Modern glass electrodes are designed to be durable and resistant to various environmental conditions.
Construction and Working of Batteries: Lead Acid Storage Battery, Ni-Cd Storage Cell, Lithium Batteries
Batteries are electrochemical devices that convert chemical energy into electrical energy through redox (reduction-oxidation) reactions. They are widely used in various applications, from powering small electronic devices to providing energy storage for electric vehicles and renewable energy systems.
Types of Batteries
Batteries can be broadly categorized into two main types: primary (non-rechargeable) and secondary (rechargeable).
-
Primary Batteries: Primary batteries are those in which only one redox reaction occurs, and the battery becomes dead after some time. These batteries are non-rechargeable, and the cell reactions are irreversible. They are designed for one-time use and cannot be recharged. Examples include:
- Alkaline Batteries: Commonly used in household devices like remotes and flashlights. They use zinc and manganese dioxide as reactants.
- Lithium Batteries: Often used in cameras and small electronics due to their high energy density and long shelf life.
- Zinc-Carbon Batteries: Inexpensive and commonly found in low-drain devices.
-
Secondary Batteries: Secondary batteries are those that recharge when an electric current passes through the cell. These batteries are rechargeable, and the cell reactions are reversible, allowing them to be used repeatedly. They can be recharged and reused multiple times. Examples include:
- Lead-Acid Batteries: Widely used in vehicles, consisting of lead dioxide (PbO₂) as the positive electrode, sponge lead (Pb) as the negative electrode, and a sulfuric acid electrolyte.
- Nickel-Cadmium (NiCd) Batteries: Known for their high discharge rates and rechargeable capabilities, often used in power tools and emergency lighting.
- Nickel-Metal Hydride (NiMH) Batteries: Common in hybrid vehicles and rechargeable batteries, with a higher capacity than NiCd batteries and less toxicity.
- Lithium-Ion Batteries: Used in mobile phones, laptops, and electric vehicles due to their high energy density, lightweight, and long cycle life.
- Solid-State Batteries: A newer technology that uses a solid electrolyte instead of liquid, potentially offering higher energy density and safety.
Lead-Acid Storage Battery
- Anode: Spongy metallic lead
- Cathode: Lead dioxide
- Electrolyte: Dilute
Construction: A number of spongy lead plates (anodes) and a number of lead dioxide () plates (cathodes) are connected in parallel. The spongy lead plates are arranged alternately between the lead dioxide plates. All the plates are separated from adjacent plates by insulators, such as wooden strips or glass fiber. The entire assembly is immersed in approximately 38% dilute with a specific gravity of 1.2 to 1.3 g/cm³.
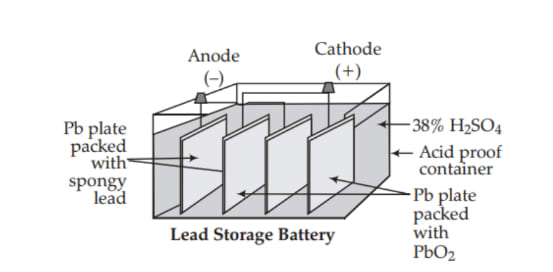
Discharging: A battery is said to be discharging when it is used to produce electrical energy. In this process, the lead electrode loses electrons and gets oxidized to , which reacts with to form . The electrons released from the anode are used by the cathode to reduce into , which also reacts with to form . During this process, deposits on both electrodes, causing the cell to stop working.
At the Anode:
At the Cathode:
Net Reaction:
Charging: A battery needs to be charged when the specific gravity of falls below 1.2 g/cm³. This can be achieved by applying an external direct current to reverse the cell reaction.
At the Cathode:
At the Anode:
Net Reaction:
Nickel-Cadmium (Ni-Cd) Storage Cell
A nickel-cadmium cell consists of two plates. The active material of the positive plate (anode) is , and the negative plate (cathode) is cadmium (Cd) when fully charged. The electrolyte is a solution of potassium hydroxide (KOH) with a small addition of lithium hydrate, which increases the capacity and life of the battery. The specific gravity of the electrolyte is 1.2.
Since the voltage produced by a single cell is low, many cells are connected in series to achieve the desired voltage output. This arrangement is known as the nickel-cadmium battery.
Nickel-Cadmium Battery Construction and Working
In these batteries, the number of positive plates is one more than that of negative plates. The container of this battery is typically connected to the negative plates.
When the cell is fully charged, its positive plate is made of nickel oxyhydroxide (NiOOH), and its negative plate is cadmium (Cd).
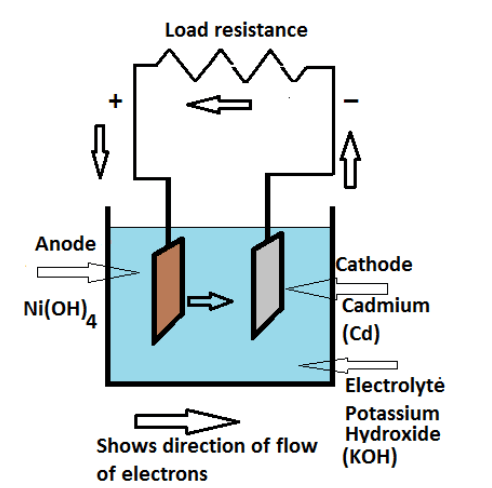
Discharging: When the cell discharges, potassium hydroxide (KOH) serves as the electrolyte but does not take part in the overall reaction. The hydroxyl () ions facilitate the redox process, moving within the electrolyte to maintain charge balance. The following chemical reactions occur:
At the Cathode (Negative Electrode - Cd):
At the Anode (Positive Electrode - NiOOH):
Thus, at the cathode, cadmium (Cd) is oxidized to cadmium hydroxide (), while at the anode, nickel oxyhydroxide () is reduced to nickel hydroxide (). The electrolyte () remains unchanged in concentration, as it only acts as an ion conductor.
Charging: When the battery is recharged, an external voltage is applied, forcing the reverse reactions to occur. Hydroxyl ions move toward the anode, while electrons flow back to the cathode, restoring the original active materials. The reactions are as follows:
At the Anode (Positive Electrode - Ni(OH)₂):
At the Cathode (Negative Electrode - Cd(OH)₂):
Thus, during charging, nickel hydroxide () is oxidized back to nickel oxyhydroxide (), and cadmium hydroxide () is reduced back to metallic cadmium (Cd). The electrolyte () continues to act as a medium for ion movement but does not get consumed in the reaction, maintaining its concentration.
Electrical Characteristics of Nickel-Cadmium Battery
- The EMF of a fully charged cell is 1.4 V, which decreases to 1.3 V rapidly. The average EMF of the cell is 1.2 V, which reduces to 1.0 V when discharged.
- The internal resistance of the cell is very low, even lower than that of a lead-acid battery. Due to this low internal resistance, they can operate at very high charge and discharge currents efficiently and safely.
- The ampere and watt-hour efficiencies of the cell are about 80% and 65%, respectively.
Advantages of Nickel-Cadmium Battery
- It has a very long active life (about 20 years).
- Since the specific gravity of the electrolyte (KOH) does not change, it can be stored indefinitely in either a discharged or charged state without suffering any adverse effects.
- It can withstand peak rates of discharge and charge of up to 20 times the normal operating rate.
- They have very low open-circuit losses.
- The impact of a high discharge rate and low operating temperature on the capacity of the battery is minimal compared to any other type of rechargeable battery.
Disadvantages of Nickel-Cadmium Battery
- It is relatively expensive.
- The EMF developed by a fully charged cell is only 1.2 V, compared to 2 V of the lead-acid cell.
- Its components are harmful to the ecosystem; therefore, its production and utilization are banned in some countries.
Lithium Batteries: Construction and Working
The rechargeable lithium-ion battery consists of one or more power-generating compartments called cells. Each cell essentially has three components: a positive electrode, a negative electrode, and an electrolyte.
The positive electrode connects to the battery's positive (+) terminal, while the negative electrode connects to the negative (−) terminal. The electrolyte, a chemical substance, is situated between them.
The positive electrode is typically made from a chemical compound called lithium cobalt oxide or lithium iron phosphate . The negative electrode is generally made from carbon (graphite), and the electrolyte composition can vary depending on the type of battery.
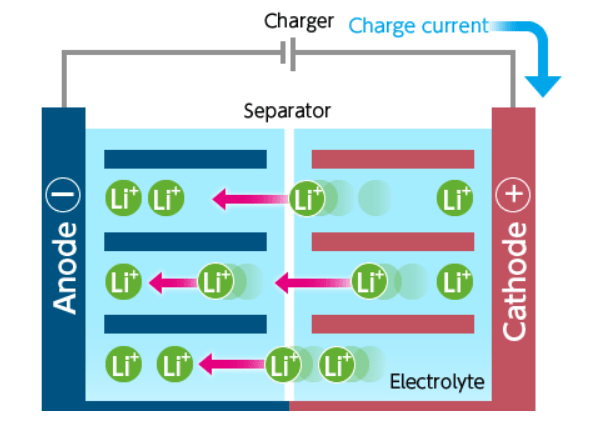
Cells with lithium anodes are called lithium cells, which are classified into two types:
- Lithium Cells with Solid Cathodes
- Lithium Cells with Liquid Cathodes
Discharging Process
When the battery discharges, lithium ions flow from the anode to the cathode through the electrolyte, releasing energy in the process. The following half-reactions occur during discharging:
At the Anode:
At the Cathode:
Charging Process
During charging, lithium ions flow from the cathode to the anode, effectively restoring the battery's charge. The following half-reactions occur during charging:
At the Cathode:
At the Anode:
Types of Lithium Cells
-
Lithium Cells with Solid Cathodes:
- Anode: Lithium
- Cathode: Manganese dioxide
- Electrolyte: A mixture of propylene carbonate and 1,2-dimethoxyethane.
Lithium-manganese dioxide is emerging as the most widely used 3-volt solid cathode lithium primary battery. The cathode should be heated to > to remove water before being incorporated into the cathode.
Anodic Reaction:
Cathodic Reaction:
Net Reaction:
Applications:
- Cylindrical cells are used in fully automatic cameras.
- Coin cells are widely used in electronic devices such as calculators and watches.
-
Lithium Cells with Liquid Cathodes:
- Anode: Lithium
- Cathode: Thionyl chloride
Anodic Reaction:
Cathodic Reaction:
Net Reaction:
Due to the nature of lithium-thionyl chloride cells, they possess very high energy density. Furthermore, the liberated as a product is liquid under the internal pressure of the cell. No co-solvent is required for the solution, as thionyl chloride is a liquid with moderate vapor pressure. The discharging voltage is between 3.3 and 3.5 volts.
Applications:
- These cells are used for military and space applications.
- They are used in medical devices such as neuro-stimulators and drug delivery systems.
- These cells are employed on electronic circuit boards to supply fixed voltage for memory protection.
Advantages of Lithium-Ion Batteries
Today, lithium-ion batteries are popular due to several important advantages over competing technologies:
- Generally, they are much lighter than other types of rechargeable batteries of the same size.
- They hold their charge well; a lithium-ion battery pack loses only about 5 percent of its charge per month.
- They provide high specific energy and high load capabilities with power cells.
- They have a long cycle life and extended shelf-life; they are maintenance-free and can handle hundreds of charge/discharge cycles.
- They offer high capacity, low internal resistance, and good coulombic efficiency.
- They have a simple charge algorithm and reasonably short charge times.
- They exhibit low self-discharge (less than half that of NiCd and NiMH batteries).
Limitations of Lithium-Ion Batteries
Despite their advantages, lithium-ion batteries also have some limitations:
- They require a protection circuit to prevent thermal runaway if stressed.
- They degrade at high temperatures and when stored at high voltage.
- Rapid charging is not possible at freezing temperatures .
- Transportation regulations are required when shipping in larger quantities.
- They are extremely sensitive to high temperatures, which can cause lithium-ion battery packs to degrade much faster than they normally would.
Fuel Cell
Fuel Cells: A fuel cell generates electrical energy without combustion from oxygen and a fuel gas that can be oxidized (such as hydrogen gas, ). Thus, a fuel cell converts the chemical energy of fuels directly into electricity. The essential process in a fuel cell can be summarized as:
In a fuel cell, one or both of the reactants are not permanently contained within the cell but are continuously supplied from an external source, while the reaction products are continuously removed. One of the most successful and simplest fuel cells is the hydrogen-oxygen fuel cell.
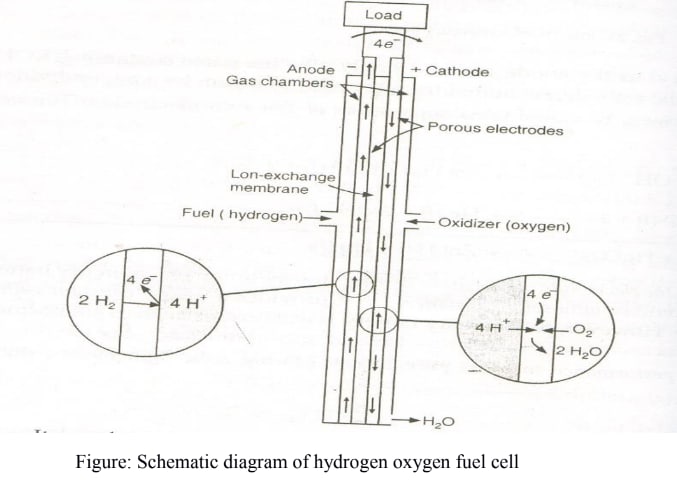
It consists of an electrolytic solution, such as 25% KOH, and two inert porous electrodes. Hydrogen and oxygen gases are bubbled through the anode and cathode compartments, respectively, where the following reactions take place:
Anode:
Cathode:
Net Reaction:
It should be noted that the only product released is . Typically, many of these cells are stacked together in series to form a battery, referred to as a fuel cell battery or fuel battery.
Advantages of Fuel Cells
- No emission of toxic gases; chemical wastes are within safe limits, making the reactants and products environmentally friendly.
- High efficiency in converting chemical energy to electrical energy, making them an excellent renewable energy resource.
- No noise pollution, unlike generators.
- Low maintenance and transportation costs for fuel.
- Compared to nuclear energy, fuel cells are economical and safe.
- Fuel cells can operate at temperatures up to 2000°C, making them suitable for high-temperature applications.
Limitations of Fuel Cells
- The main limitation lies in the high initial costs associated with electrode materials and design.
- Large weight and volume of hydrogen gas fuel storage systems.
- High cost of hydrogen gas.
- Lack of infrastructure for the distribution and marketing of hydrogen gas.
- Most alkaline fuel cells suffer from gas leakage.
Solar Cell
A solar cell in electrochemistry is a device that directly converts light energy into electrical energy through a process known as the photovoltaic effect. It is essentially a type of photoelectrochemical cell. The most common type of solar cell is made from semiconductor materials, typically silicon.
-
Photovoltaic Effect:
- In a solar cell, photons from sunlight strike the surface of a semiconductor material, like silicon.
- When the photons possess enough energy, they excite electrons, causing them to jump from the valence band to the conduction band, creating electron-hole pairs.
-
p-n Junction:
- Solar cells often involve a p-n junction, where one side of the semiconductor is doped with a material that creates an abundance of positive charge carriers (holes), while the other side is doped with a material that provides extra electrons.
- The electric field at the p-n junction separates the electrons and holes, generating a flow of current when the cell is connected to an external circuit.
-
Electron Flow:
- Electrons are directed towards an external circuit, creating an electric current that can power devices.
- The overall electrochemical reaction in a solar cell is simply the conversion of light energy into electricity, without any chemical byproducts like those found in fuel cells or batteries.
Working of a Silicon Solar Cell
-
At the Anode (n-type silicon): The sunlight excites electrons, freeing them to move towards the anode (negative terminal).
-
At the Cathode (p-type silicon): The freed electrons move through the external circuit towards the cathode (positive terminal), completing the circuit and generating electrical energy.
Key Equation (Photovoltaic Cell Efficiency)
The efficiency of a solar cell is given by the ratio of electrical power output to the incident light power:
Where,
Applications
- Solar cells are widely used in solar panels to generate clean, renewable energy from sunlight.
- They have applications in calculators, satellites, and solar farms, contributing to sustainable energy solutions.
Advantages of Solar Cells
- Solar energy is abundant and renewable, making it a sustainable option compared to fossil fuels.
- Solar cells do not emit greenhouse gases or pollutants, reducing environmental impact.
- Once installed, solar cells require minimal maintenance, and the energy they produce is free.
- Solar cells enable decentralized power generation, reducing dependence on external energy supplies.
- Solar panels can be used for small applications (like calculators) or scaled up for large solar farms.
- Solar cells generate electricity without noise, making them suitable for residential and urban areas.
- Many governments provide tax incentives, rebates, or subsidies for solar installations.
Limitations of Solar Cells
- Solar cell efficiency decreases in cloudy or rainy weather and is ineffective at night.
- The upfront cost of purchasing and installing solar panels can be high, though prices have been decreasing.
- Large-scale installations require significant land or rooftop space to be effective.
- Solar energy needs to be stored (usually in batteries) for use during non-sunny periods, which adds cost and complexity.
- Current solar cells have limited efficiency, typically converting 15-20% of sunlight into electricity.
- The manufacturing process for solar cells involves the use of rare and sometimes hazardous materials.
- Solar energy is more effective in areas with high sunlight exposure, making it less viable in regions with low sunlight.
Aspect | Solar Cell | Fuel Cell |
---|---|---|
Energy Source | Sunlight (photovoltaic effect) | Chemical reaction between fuel (e.g., hydrogen) and oxygen |
Working Principle | Converts light energy directly into electrical energy | Converts chemical energy into electrical energy through oxidation of fuel |
Reactants | No fuel required, only sunlight | Requires continuous supply of fuel and oxygen |
By-products | None, except electricity | Water, heat, and other reaction products depending on the fuel used |
Environmental Impact | No emissions; clean energy | Clean energy (if using hydrogen); some fuels may produce emissions (e.g., natural gas) |
Energy Storage | Requires battery storage for nighttime or low-light use | No storage required; as long as fuel is supplied, energy is generated |
Lifetime | Solar panels degrade over time, typically 20-30 years. | Fuel cells last as long as fuel is supplied, with periodic maintenance |
Installation | Fixed installation (rooftops, fields) | Can be portable, depending on the design |
Dependency | Weather-dependent, requires sunlight | Fuel-dependent, requires continuous supply of fuel |
Use in Space | Used for satellites, space stations, and space missions | Used in space missions (e.g., hydrogen fuel cells in space shuttles) |