X-rays
Topic asked in Applied Physics 2023 (CBCS/NEP) question paper Section C - 6(a).
X-rays are a form of electromagnetic radiation, similar to visible light but with much higher energy. They have very short wavelengths, typically ranging from about 0.01 to 10 nanometers, which allows them to penetrate various materials, including human tissues. This property makes them useful for a variety of applications, particularly in medicine and industry.
X-rays Production
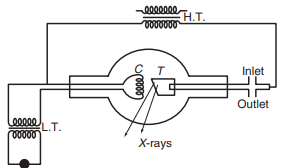
X-rays are produced when high-speed electrons strike a target made of a material with a high melting point and high atomic number, such as tungsten, platinum, molybdenum, copper, or chromium. A common device used to produce X-rays is the Coolidge tube (check fig above). This tube is evacuated to a very low pressure (around mm Hg) to create a vacuum.
Inside the tube, there is a cathode and a target. The filament of the cathode is heated with a low-voltage source to emit electrons. A high voltage (typically between 10,000 and 30,000 volts) is then applied between the filament and the target to accelerate these electrons. When the high-speed electrons collide with the target material, X-rays are generated. The wavelength of the X-rays produced depends on factors such as the nature of the target material and the initial and final energies of the incident electrons.
Only about 0.2% of the energy of the electron beam is converted into X-rays; the rest is transformed into heat. To manage this heat, the target is mounted on a hollow copper tube through which cold water circulates.
Control of Intensity: The intensity of the X-rays can be controlled by adjusting the current in the filament, which in turn controls the number of electrons striking the target. A higher current results in a greater number of incident electrons and thus more intense X-rays.
Control of Penetrating Power: The penetrating power of X-rays is controlled by adjusting the potential difference (voltage) between the filament and the target. Increasing the voltage increases the energy of the incident electrons, which produces X-rays with higher energy and greater penetrating power.
X-rays Properties
- Electromagnetic Radiation: X-rays are electromagnetic radiation with very short wavelengths, typically (0.01 to 10 nanometers), and they travel at the speed of light.
- Behavior in Fields: X-rays are not deflected by electric and magnetic fields, which indicates that they are uncharged particles and thus behave differently from charged particles like electrons.
- Fluorescence: X-rays can produce fluorescence in certain substances, such as barium platinocyanide, zinc sulfide, and others. This property is utilized in X-ray imaging and detection.
- Photographic Effect: X-rays can affect photographic plates, and their effect is often more intense than visible light due to their higher energy.
- Ionization: X-rays ionize gases when they pass through them. This means they can create ions by removing electrons from atoms or molecules in the gas.
- Photoelectric Effect: X-rays can produce the photoelectric effect, where photons of X-rays eject electrons from atoms in a material.
- Penetration: Due to their high energy, X-rays can pass through many solids, making them useful for imaging and inspection purposes.
- Interference, Diffraction, and Polarization: X-rays can exhibit wave-like behaviors such as interference, diffraction, and polarization, similar to visible light. These properties are used in various scientific techniques, including X-ray crystallography.
Hard and Soft X-rays
Hard and soft X-rays refer to two different types of X-rays that differ primarily in their energy levels and penetrating power. Here’s a breakdown of the differences:
Hard X-rays
- Energy: Hard X-rays have higher energy, typically ranging from about 30 keV (kilo-electron volts) to several hundred keV.
- Wavelength: They have shorter wavelengths, generally between 0.01 and 0.1 nanometers.
- Penetrating Power: Because of their higher energy and shorter wavelength, hard X-rays have greater penetrating power. They can pass through dense materials like metals and bones, which makes them useful in industrial inspections and medical imaging, such as in CT scans.
- Applications: Hard X-rays are used for detailed imaging and analysis in fields such as radiography, industrial inspection, and crystallography.
Soft X-rays
- Energy: Soft X-rays have lower energy, typically ranging from about 0.1 keV to 30 keV.
- Wavelength: They have longer wavelengths, generally between 0.1 and 10 nanometers.
- Penetrating Power: Due to their lower energy and longer wavelength, soft X-rays have lower penetrating power. They are absorbed by less dense materials and are less effective at penetrating through thick or dense substances.
- Applications: Soft X-rays are used in applications that require imaging or analysis of less dense materials, such as in certain biological studies, soft tissue imaging, and materials science research.
In summary, hard X-rays are more penetrating and are used for imaging and analysis of dense materials, while soft X-rays are less penetrating and are suited for studying less dense materials.
Continuous and Characteristics X-rays
X-ray spectra consist of two types of spectra, namely continuous spectrum and characteristic or line spectrum.
Continuous X-ray Spectrum
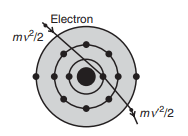
The continuous X-ray spectrum is produced when a sufficiently high potential is applied between the target and filament. In this process, some of the high-speed electrons penetrate the core of the atoms in the target material (as shown in fig above). As these electrons are decelerated upon interacting with the target material, they lose kinetic energy. According to classical theory, a decelerated charged particle emits energy in the form of X-rays. This results in a range of wavelengths being emitted, creating a continuous spectrum.
Relationship Between Frequency and Kinetic Energy
The relationship between the frequency () of the emitted X-ray photon and the change in kinetic energy of the incident electron can be expressed as:
where is the initial velocity of the electron and is the reduced velocity after deceleration.
If the incident electron is completely stopped, the frequency of the emitted X-ray photon reaches its maximum value. This can be given by:
where is the accelerating potential applied to the electron. The corresponding minimum wavelength () of the continuous X-ray spectrum can be determined using:
Since,
equation 1 becomes,
or,
where is Planck’s constant, is the speed of light, and is the elementary charge.
Substituting the values for , , and , the minimum wavelength can be calculated as:
where is in volts.
Features of the Continuous X-ray Spectrum
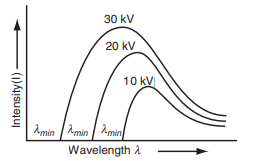
- Production Mechanism: The X-rays are produced due to the deceleration of fast-moving electrons in the target material.
- Intensity: The intensity of the continuous spectrum increases as the accelerating potential is increased (fig above).
- Wavelength Limit: The minimum wavelength limit () shifts toward shorter wavelengths as the accelerating potential is increased, reflecting the inverse proportionality between and .
Characteristic X-ray Spectrum
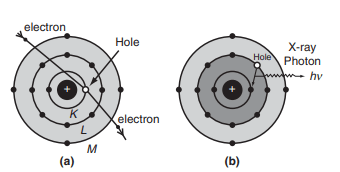
The characteristic X-ray spectrum consists of well-defined wavelengths superimposed on the continuous spectrum. The spectral lines typically occur in small groups. The characteristic X-ray spectrum is produced when extremely high-energy electrons penetrate deep inside the atoms of the target material and collide with tightly bound electrons in the innermost K shell (orbit) of the atom as shown in fig above.
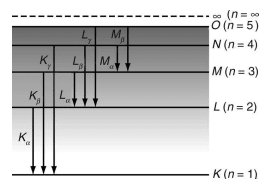
When an electron from the K shell is ejected, a vacancy is created. This vacancy can be filled by an electron from a higher energy level, such as the L or M shell. If an electron from the L shell fills the vacancy, a line is produced. If an electron from the M shell fills the vacancy, a line is produced, and so on. This process results in different series such as K, L, M, etc., in the emission of X-ray spectra (fig above).
The energy of the emitted X-ray photon corresponding to the line is given by:
where and are the binding energies of the electrons in the K and L shells, respectively.
Below is a plot (fig.) depicting the intensity versus wavelength for characteristic X-ray spectrum superimposed on the continuous spectrum.
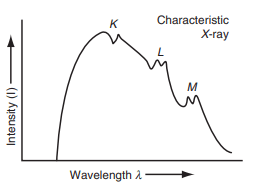
Features of Characteristic X-ray Spectrum
- Line Spectrum: The line spectrum is produced when electrons from the innermost orbit are ejected, followed by electrons from outer orbits filling the vacancies.
- Wavelength Shifts: The wavelength of the lines in the K-series shifts towards lower values (higher energy) as the atomic number of the target element increases.
- Intensity of Spectral Lines: The intensity of a specific spectral line depends on the probability of that particular electronic transition.
Bremsstrahlung Effect
Topic asked in Applied Physics 2023 (CBCS/NEP) question paper Section E (Compulsory) - 9(b).
Bremsstrahlung, which is German for "braking radiation," refers to the radiation emitted by charged particles, typically electrons, when they are decelerated or deflected by other charged particles, usually nuclei. This effect is a major source of continuous X-ray spectra.
Mechanism of Bremsstrahlung
When a high-speed electron passes near the nucleus of an atom, it experiences a strong electric field due to the positive charge of the nucleus. This interaction causes the electron to decelerate and change direction. The loss of kinetic energy from the electron is emitted in the form of X-ray photons. The energy and wavelength of the emitted X-rays vary, resulting in a continuous spectrum.
The spectrum and intensity of Bremsstrahlung radiation can be described by complex formulas, but the general trend is that the intensity of the emitted radiation is inversely proportional to the wavelength , and it increases with the energy of the incident electron. For a given electron energy, the total power radiated as Bremsstrahlung can be approximated by:
where:
- is the atomic number of the target material,
- is the charge of the electron,
- is the speed of light,
- is the energy of the incident electron,
- is the mass of the electron.
Features of Bremsstrahlung
- Continuous Spectrum: Unlike characteristic X-rays which have discrete lines, Bremsstrahlung produces a continuous spectrum of X-ray wavelengths.
- Intensity: The intensity of Bremsstrahlung increases with the atomic number of the target material and the energy of the incident electrons.
- Dependence on Electron Energy: Higher energy electrons will produce higher energy (shorter wavelength) X-rays.
- Penetrating Power: X-rays produced by Bremsstrahlung have high penetrating power and can pass through various materials.
References: Engineering Physics, H.K Malik & A.K Singh, Tata McGraw-Hill.